Overview
The Brozek Lab is a group of synthetic chemists making new materials capable of energy capture, catalysis, and novel electronic properties. Molecular inorganic and organic chemistry forms the basis of how we build new materials and how we think about chemical and physical problems.
We synthesize "soft materials", such as MOFs, colloidal nanocrystals, clusters, and ionic liquids, that behave as both small molecules and extended solids, and study their redox chemistry through physical inorganic methods. Current targets include nanocrystals, porous semiconductors, and ionic liquids.
In addition to studying these areas, students acquire training in air-free synthesis, spectroscopy, electrochemical techniques, and solid-state characterization.
A New Class of Semiconductor Nanocrystals

Nanocrystals of metal–organic frameworks are used industrially as membranes for some of the largest separation processes in the world, where nanocrystal sizes and morphologies exert a profound influence on separation performance, and yet, controlling MOF nanocrystal growth remains an open research frontier. The Brozek lab has pioneered the development of synthetic methods and models to achieve reproducible control over MOF particle sizes and colloidal stability. Breakthroughs in our lab have led to the first examples of colloids that can be characterized by solution-state techniques, revealing fascinating size-dependent properties, and easily processed into functional thin films for wide-ranging technologies.
Water Purification Membranes
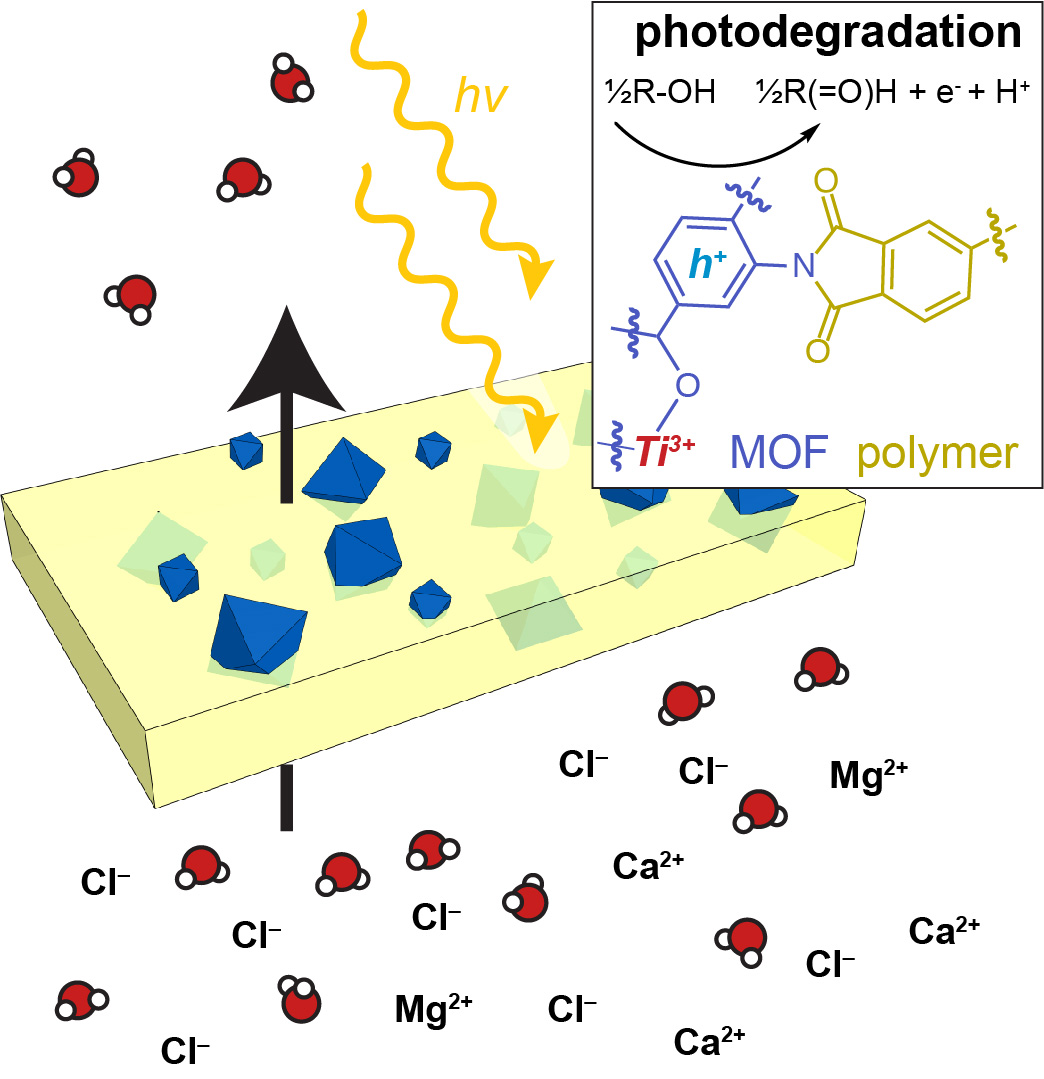
We investigate water purification membranes that simultaneously accomplish the goals normally meant for many separate water filtration steps – toxin degradation and ion capture of contaminants at sizes ranging from centimeters to sub-nanometers. Because our membranes incorporate nanoparticles with the highest known surfaces of any material and with diverse compositions that can be tuned by molecular chemistry, they stand to achieve greater selectivity and efficiencies than current water purification materials, while providing insight into fundamental chemistry of a new class of nanomaterial.
Sustainable Energy Storage

Charge transport and ion transport mechanisms dictate the performance of batteries, fuel cells, capacitors, and near all electrochemical energy technologies. The Brozek lab is pioneering research into the coupled movement of ions and charges, with particular emphasis on transport mechanisms in porous energy electrodes, where ion-coupled charge transfer is especially relevant. Porous conductors are envisioned as advanced electrodes and membranes because their high surface areas and tunable compositions allow superior transport and storage properties. We leverage insights into ion-coupled charge transport to design next-generation energy technologies.
Self-Healing Materials

Materials are typically assumed to be structurally static, but many of the most important physical properties of molecules and materials arise from dynamic motion. Virbonic interactions, reversible bonding, and stimuli-responsive behavior invovle bonds breaking and reforming in reversible equilibrium. Self-healing properties, phase-change behavior, and countless material applications emerge from dynamic bonding in materials. The Brozek lab has prioneered the study of dynamic bonding in framework materials, including MOFs, perovskites, and Prussian Blue analogs. We discovered some of the first evidence of dynamic bonding in MOFs and continue to research how reversible structural distortions help explain optical, catalytic, magnetic, and electronic applications of frameworks.